The theory was that because of evolution, the human brain consists of the reptilian complex (basal ganglia or R-complex: reptilian brain or lizard brain), the paleomammalian complex (limbic system: amygdalae, hypothalamus, hippocampal complex, and cingulate cortex), and the neomammalian complex (neocortex). Each were viewed as independently conscious structures sequentially added to the forebrain in the course of evolution. This notion of the Triune Brain was put forth by the American physician and neuroscientist Paul D. MacLean in the 1960s. When he observed that parts of the mammalian brain were very similar to parts of the reptilian brain, he concluded that the brain had evolved in stages after the first tetrapods (four limbed vetebrates) crawled out of the water on to land 320 million years ago, with the reptilian brain developing first, followed by the limbic system. With the rise of the primatesm the neocortex evolved over all of them. Each of these sections are responsible for different functions, with the basal sections and limbic system involved in survival instincts such aggression, territorial and ritual displays, reproduction, feeding and parental behaviour. According to MacLean, the neocortex was the most recent step in the evolution of the mammalian brain and this is the section is responsible for the ability for language, abstraction, planning, and perception.
However, ever since the 1970s, this concept has been the subject of criticism and is generally regarded as a myth. But because it survived in popular consciousness for so long, the triune brain idea has also been called “one of the most successful and widespread errors in all of science.” It is now generally accepted that this is not how the brain works.
When the first tetrapods left their watery world for land 320 million years ago, evolution took a major turn and eventually the Earth was full of three clades of animals: reptiles, birds and finally mammals. Because they all have common ancestry, the brains of all tetrapods share similarities. It is however unclear how variations in the brain contributed to clade-specific attributes. A new study sought to understand the underpinnings of the interconnecting brain regions and how neural networks worked together.
The team of researchers from the Max Planck Institute for Brain Research looked at a dragon brain and compared it with one from a mouse to find answers and published their results in a paper in the journal Science. They did this by generating and comparing molecular structures of both brains.
“Neurons are the most diverse cell types in the body. Their evolutionary diversification reflects alterations in the developmental processes that produce them and may drive changes in the neural circuits they belong to”, says Prof. Gilles Laurent, Director at the Max Planck Institute for Brain Research who led the new study. “For example, distinct brain areas do not work in isolation, suggesting that the evolution of interconnected regions, such as the thalamus and cerebral cortex, might in some way be correlated. Also, a brain area in reptiles and mammals that derived from a common ancestral structure might have evolved in such a way that it remains ancestral in one clade today, while it is “modern” in the other. Conversely, it could be that both clades now contain a mix of common (ancient) and specific (novel) neuron types. These are the sorts of questions that our experiments tried to address”, Laurent adds.
Because traditional approaches to compare the developmental regions of the brain were not sufficient to get full details of the similarities and differences, Laurent and his team used a technique called single-cell RNA sequencing (RNA is a single strand messenger molecule used to form proteins and for other cellular functions). This technique detects a large fraction of the RNA molecules (transcriptomes) present in single cells. The researches then generated a cell-type atlas of the brain of the Australian bearded dragon Pogona vitticeps and compared it to existing mouse brain datasets.
“We profiled over 280,000 cells from the brain of Pogona and identified 233 distinct types of neurons”, explains David Hain, graduate student in the Laurent Lab and co-first author of the study. “Computational integration of our data with mouse data revealed that these neurons can be grouped transcriptomically in common families, that probably represent ancestral neuron types”, says Hain.
What this means is that mammals and reptiles had an initial set of neurons with similar transcriptomes that they both have in common, even after millions of years of evolution. But contrary to popular belief, these neurons are not restricted to just the reptilian region of the brain. In fact, most regions of the brain have these ancestral neurons, as well as newer types.
“Multiple brain areas contain mixtures of similar and divergent neurons, suggesting ubiquitous neuron diversification in these brain regions,” according to the statement. Basically, most areas of the brain contain a mix of common (ancient) and specific (novel) neuron types, as shown in the figure below.
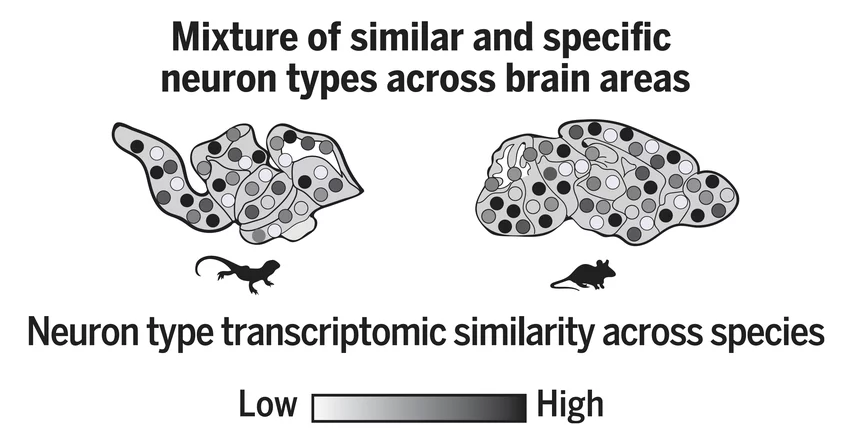
Reptiles and mammals are evolutionary separated by over 300 million years. Max Planck scientists generated a cell type atlas from the brain of a lizard. Computationally integration of this data with mouse transcriptomics revealed that multiple brain areas contain mixtures of similar and divergent neurons, suggesting ubiquitous neuron diversification in these brain regions. © Max Planck Institute for Brain Research / G. Laurent; Hain et al. Science 2022 DOI: 10.1126/science.abp8202
This negates the idea that some parts of the brain are older than the others.
Furthermore, “neurons in the thalamus could be grouped in two transcriptomic and anatomical domains, defined by their connectivity to other regions of the brain. Because these connected regions have had different fates in mammals and in reptiles, one of these regions being highly divergent, comparing the thalamic transcriptomes of these two domains proved to be very interesting. Indeed, it revealed that transcriptomic divergence matched that of the target regions”, according to the statement. That is, the transcriptomes diverged in such a way, they matched the region to which they were connected.
“This suggests that neuronal transcriptomic identity somewhat reflects, at least in part, the long-range connectivity of a region to its targets. Since we do not have the brains of ancient vertebrates, reconstructing the evolution of the brain over the past half billion years will require connecting together very complex molecular, developmental, anatomical and functional data in a way that is self-consistent. We live in very exciting times, because this is becoming possible” concludes Laurent.
The research has been published in Science.